Understanding space in laboratory
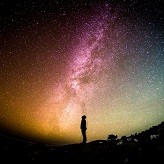
Their dimensions and distances make most astrophysical phenomena inaccessible to experimentation. To better understand them, researchers use laboratory astrophysics, that is to say, they reproduce on a small scale the conditions in which different phenomena are formed or evolve.
This month, the prestigious scientific journal Nature Communication published two studies from the Laboratory pour l'utilisation des lasers intenses (Intense lasers laboratory LULI*), one devoted to the shape of astrophysical plasma jets, and the other to the properties of materials located at the heart of exoplanets.
Turbulent plasma jets
Astrophysical jets are spectacular sights: they are extremely long elongations plasma emerging from young stars, black holes and even our Sun.
Most of the time, these “flames” are not continuous, like a gas stove for example. Their emission is turbulent and the plasmas have curves and knots, “like a string of morse-code or the emission of an old diesel car” explains Julien Fuchs, CNRS research director at LULI.
These irregularities were speculated to be from changes in the structure of the surrounding magnetic field, but up to now, there was no way of experimentally verifying this hypothesis.
Julien Fuchs has been working on experimental techniques to reproduce these plasma jets in the laboratory for several years, and was awarded an ERC Advanced grant in 2018 for his GENESIS project dedicated to the further development of this discipline.
"A few years ago, we established an experimental technique that was able to re-create centimeter-long plasma jets in the laboratory that closely matched the characteristics of jets created by young stars," explains the researcher. By irradiating a piece of plastic with a high-powered laser, immersed in a magnetic field aligned parallel to the laser beam, they were able to reproduce an extremely high velocity jet guided by the magnetic field.
Their latest study, carried out with several European collaborators and published in Nature Communications, focused on the influence of the direction of the magnetic field on the plasma. “We introduced an angle between the laser-created plasma and the ambient magnetic field,” explains Julien Fuchs. “We found that indeed there was a disruption in the collimation of the jet and plasma leaking through the magnetic field lines.”
Knowing that the magnetic fields surrounding astrophysical jets can change during their lifetime, observations of irregularities in the jet would not be unusual. Nevertheless, by coupling experimental observations with simulations, researchers have shown that one possible explanation for the observed "morse code" jet structure is that they are induced by the ever-changing nature of local magnetic fields.
Magnetic fields in super-Earths
The discovery of more than 4,000 planets outside our solar system raises the exciting promise of discovering some of them capable of hosting life. Researchers are particularly interested in planets with masses between one and a few Earth masses. These "super-Earths" are supposed to be rocky, with a core of iron and nickel, and a mantle (the part surrounding the core) of silicates.
The existence of a magnetic field on a planet is considered to be one of the necessary conditions for the presence of life. Protecting the globe from cosmic radiation, it can be generated inside a planet when it has a conductive fluid animated by convection movements. By rotating on itself, this fluid will produce a magnetic field, as it is the case for our planet: the liquid part of its iron core is animated by convection movement.
In the case of super-Earths the iron core is probably entirely crystallized because of the strong gravity (these planets are more massive than ours), which would prevent the existence of a magnetic field. An alternative considered by scientists could be the generation of the magnetic field in the mantle of electrically conductive fused silicates.
To determine whether this possibility is valid, it is necessary to be able to measure the electrical conductivity of these materials under the conditions that prevail in the heart of the super-Earths, i.e. temperatures of several thousand degrees and pressures several million times that of our atmosphere.
A research team at LULI, in collaboration with CEA, has studied the properties of silicates by experimentally reproducing these extreme conditions. This work was published this month in Nature Communications and shows that the conductive mantle hypothesis seems viable.
"Experimentally reproducing these conditions is very complex" explains Alessandra Ravasio, CNRS researcher at LULI. "We have compressed quartz (SiO2) samples by generating two successive shock waves using the LULI2000 laser at the laboratory," she describes. "Contrary to compression by a single shock that generates very high temperatures, the double shock technique allowed us to generate high pressures, while keeping relatively moderate temperatures, more relevant to the interiors of super-Earths. ».
This innovative compression scheme allows to study the properties of compressed material under a vast set of thermodynamic conditions that have not been explored until now. The researchers thus applied this double shock method to their quartz samples and, by coupling experimental data to ab initio calculations, they were able to estimate the electrical conductivity of SiO2.
Their methodology allowed them to take a new approach, without resorting to commonly used models whose validity in this context was questioned. "Our results indicate electrical conductivities for SiO2 that are high enough to sustain magnetic fields inside super-Earths," explains Alessandra Ravasio.
This confirmation will allow astrophysicists to improve their models and better guide the study of potentially habitable exoplanets.
*LULI: a joint research unit CNRS, École Polytechnique - Institut Polytechnique de Paris, CEA, Sorbonne Université