Quantum entanglement: from a philosophical debate to the Nobel Prize
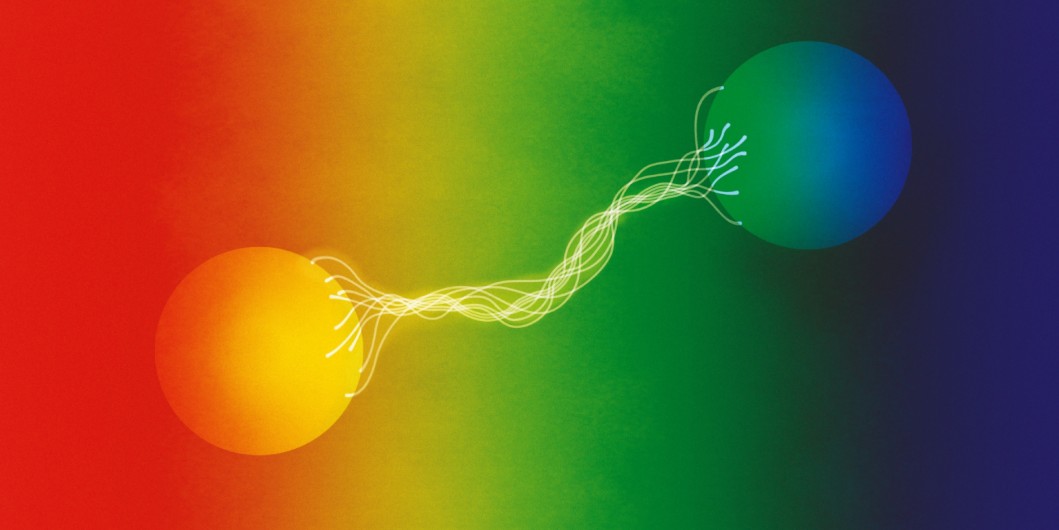
Can quantum mechanics be considered complete? This was the question raised in a scientific article published in 1935 by Albert Einstein and his collaborators Boris Podolsky and Nathan Rosen. Is there not something missing in this theory to make it a complete description of the world, as Einstein claimed in a debate against the Niels Bohr at the time? Experiments conducted from the 1970s onwards, notably by Alain Aspect, John Clauser and Anton Zeilinger, have provided answers to this seemingly abstract question. This earned these three scientists the 2022 Nobel Prize in Physics.
A thought experiment
In 1935, quantum physics was a recent theory - it was formalised in the 1920s - but already powerful. It made it possible to understand many phenomena at the microscopic scale, but also at our scale, such as the fact that matter is stable, as well as the very concrete characteristics of materials: how they conduct electricity, what light they can emit or absorb, etc. This led shortly afterwards to the 'first quantum revolution' (the invention of the transistor, for example). Unlike classical physics, quantum physics places probabilities at the heart of its formalism.
Albert Einstein did not question the results or predictions of this theory, which he himself helped to develop. However, he believed that quantum physics was not the ultimate level of description of reality and that a more detailed theory would, for example, make probabilities less fundamental. Niels Bohr did not agree. The two scientists exchanged arguments through 'thought experiments', involving the manipulation of particles one by one (which was not possible in practice back then). The 1935 article proposes such a thought experiment.
In order to understand the problem, let us imagine for example two particles located in a box. Two colours are possible for these particles: white or black. Intuitively, one might think that there are four options: both particles are white; both particles are black; the first is white and the second is black; the first is black and the second is white. But the formalism of quantum physics also allows for mixtures of these four options, what physicists call superpositions of states. For example, that the two particles are both in the state where the first is white and the second is black and in the state where the first is black and the second is white, without it being possible to distinguish between the two unless an observation is made. Such a state is called "intricate". It is represented with the balls striped with a question mark in the following figure.
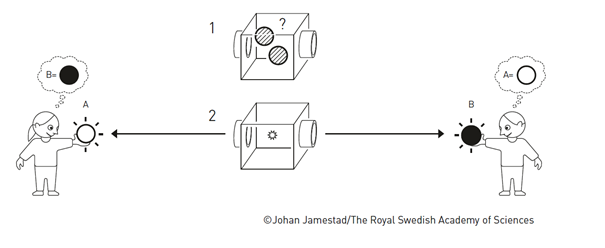
In quantum physics, two objects can be 'entangled'. If we send each of them to different locations, we observe correlations between their properties (in this case, the colour black or white), without it being possible to assign a specific colour to each object before the observation. This situation goes against our common intuition.
This is one of the things that particularly bothered Albert Einstein: imagine a system that sends one of these two entangled particles to physicist A and the other to physicist B. If physicist A observes that the particle she has received is white, then the particle received by physicist B is necessarily black. If physicist A observes that the particle she has received is white, then, necessarily, the particle received by physicist B is black. There is a correlation between the results. What contradicts the intuition is that this colour seen by B was not determined before the observation made by physicist A. This observation seems to have influenced, at a distance and without interaction, the result of the observation of physicist B. This 'non-local' correlation made Einstein think that something was missing in the quantum description.
Indeed, there may be a more intuitive explanation. After all, correlations are not unusual in everyday life. Take a black ball and a white one, mix them up without looking at them. Give one ball at random to physicist A who goes to Paris and one to physicist B who goes to Marseille. Of course, if physicist A observes that the ball she received is white, then, of course, the ball received by physicist B is black. Nothing surprising: each marble had a specific colour from the start. What if the 'non-local' correlations linked to entanglement were in fact only the usual correlations, and that hidden variables - i.e. parameters that would still escape the quantum formalism - determined the results of the observations from the start?
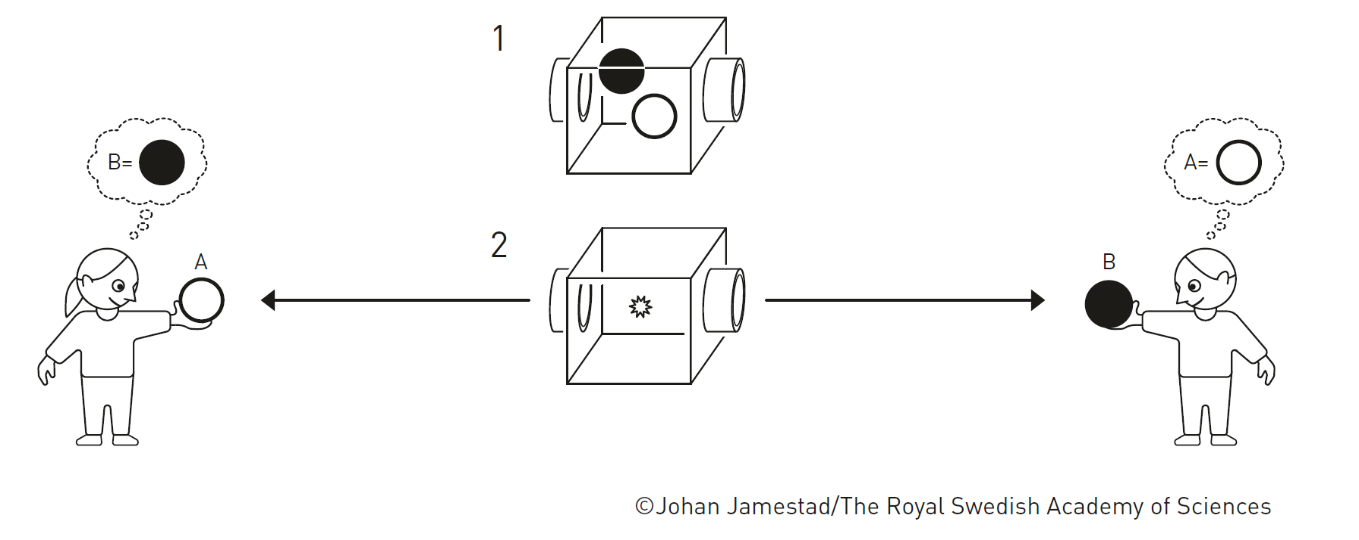
The same correlations as in the figure above would be more intuitive if, from the start, a well-defined colour was assigned to each object, even if no observer knew about it before the measurement. In this case, "hidden variables" (inaccessible to the observer) would explain the result.
The debate turns into an experiment
The debate between Bohr and Einstein did not particularly interest the scientists of the time. After all, in both cases, the observed results are the same and quantum physics remains effective. At most, it was a philosophical choice. In 1964, however, John Bell showed in a theoretical paper that certain experimental configurations with entangled particles would give different observations depending on whether or not there were local hidden variables. These demarcation criteria are called Bell's inequalities. In 1969, John Clauser, Michael Horne, Abner Shimony and Richard Holt reformulated these inequalities and showed that it is possible to test them with existing technologies using photons, particles of light, which can be entangled. In other words, it becomes possible to experimentally settle the past debate between Einstein and Bohr.
In the 1970s, several experimentalists, including John Clauser in the United States and Alain Aspect in France at Institut d'Optique Graduate School, started to investigate this question. Their experiments are based on the principle of the examples shown above, where the roles of the physicist are played by detectors A and B. In order to test Bell's inequalities, it is necessary to measure the polarisation of the photons (a property that plays the role of the colour of the ball in the example given above), using several polarisers oriented in different directions. In order for the test to be ideal, measurements at A must also be independent of those at B. Otherwise, there could, for example, be an as yet unknown interaction that would send a signal to the detectors, which would determine the results even before the photons arrive. Assuming that such a signal could not travel faster than light, it must be possible to choose the orientation of the polarizers in a time shorter than that taken by light to travel between the detectors. This last point is the tour de force of the experiment by Alain Aspect and his collaborators Phillipe Grangier, Gérard Roger and Jean Dalibard: the orientation of the polarisers changed every 10 nanoseconds, whereas a possible interaction signal would have taken 40 nanoseconds to cross the 12-metre space between the detectors.
Verdict and technological applications
In 1982, Alain Aspect's results were clear: Bell's inequalities were not respected, there were no local hidden variables that could explain the observed correlations. Quantum physics stood the test. Further experiments refined the result, in particular those of Anton Zeilinger, which excluded other possible flaws in their interpretations. Anton Zeilinger and his team also demonstrated the - misnamed - quantum teleportation. Since then, these properties of superposition and entanglement have been studied in detail in multiple systems (it is for example possible to entangle many particles or sets of particles). This has also launched what some, including Alain Aspect, call the "second quantum revolution", which exploits these properties as resources for secure communications, ultra-sensitive detectors or a possible future quantum computer.
Was Albert Einstein wrong? It's hard to say yes. On the one hand, he had the merit of pointing out a subtle problem in quantum physics that has proved very fertile. On the other hand, the epistemological debates on the interpretation of quantum physics are far from over (hypotheses of superdeterminism, non-local hidden variables...). But one thing is for sure: this is one of the richest intellectual and scientific adventures of the 20th century.
More information :