A nuclear safety issue: Modeling corium fusion
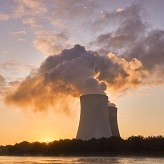
While severe accidents are extremely rare in nuclear power plants, it is nonetheless vital to be able to prevent them and, if they do occur, to manage them rigorously to contain their consequences.
This is why physicists Mathis Plapp, CNRS research director and Hervé Henry, CNRS researcher, both from the Physics of Condensed Matter Laboratory (PMC*), are working in collaboration with Romain Le Tellier (CEA Cadarache) on modeling certain aspects of severe nuclear reactor accidents, with a long-term perspective of prevention. They are particularly interested in the modeling of corium, a mixture that forms during the melting of a reactor core after a cooling failure. This phenomenon occurred at Chernobyl in 1986.
Interview with Mathis Plapp and Hervé Henry
Mathis Plapp, originally, you are a fundamentalist physicist. What led you to work on nuclear energy?
Mathis Plapp: I specialize in the formation of microstructures during the solidification of steel, a subject at the frontier between fundamental and applied research. If, with Hervé Henry, we looked into nuclear energy, it was to answer questions from our colleague Romain Le Tellier, from the Commissariat à l'énergie atomique et aux énergies alternatives (CEA) in Cadarache. These questions have extremely sensitive practical consequences, but they are also very stimulating for research.
Corium is a liquid mixture that is formed during the melting of the core of a nuclear reactor when the temperature exceeds 2500°C. It is a metallic and mineral magma that resembles an alloy in some aspects. But it has the particularity of being self-heating.
This does not mean, let's be clear, that the fission reaction is expanding. But it is not finished. And corium, which contains extremely radioactive heavy metals, can "melt" its way to the water table and contaminate everything.
Corium was formed, we know for sure, during the accidents at Three Mile Island (Pennsylvania, 1979) and Chernobyl (USSR, now Ukraine, 1986). And perhaps in Fukushima (Japan, 2011).
In the absence of direct observations, how does one represent a phenomenon such as the formation of corium?
Hervé Henry: In the case of serious accidents, we have to go through modelling. These methods are developing a lot today, both because of the power of numerical tools, but also because it meets a real need, particularly in industries that are developing new alloys (for aircraft engines, for example).
But this is not limited to metals. Numerical simulation in materials is a megatrend. We can speak of a global movement, with the emergence of computational material science. The development of reliable numerical models saves time and money.
But corium is a borderline case, because we don't have the means to test hypotheses. The fundamental equations of physics involved in its formation are known, so the challenge is to find new laws. But the application to concrete materials, and in particular to the composite magma that is corium, is very complicated.
Mathis Plapp: The "phase field" method, which is used in a lot of work on microstructures, is well suited to dealing with this subject. You have to imagine the material: very heterogeneous, with crystalline "grains" that germinate by aggregating molecules, and between these grains there are joints that are as many interfaces. This microstructure is decisive for the properties of the material.
The phase field method describes the interfaces and their growth. It is based on a thermodynamic approach, with different phases (liquid, solid, gaseous): for a given point, do we find solid or liquid? It depends on density, temperature, other parameters such as the thickness of the interface, or the coexistence of ordered phases with disordered phases.
The equations that describe and model these magma evolutions are not linear. There is no real method to "solve" them, but they can be set in a computer. You can write a simulation program from phenomena that you know well at the macroscopic level, and whose parameters you know. This will be used to simulate and explore phenomena that we know little about.
But you evoked a self-heating phenomenon. Aren't we getting out of the usual patterns?
In any crystallization, there is latent heat, which can be explained by the laws of thermodynamics. In the case of corium, there is a heating effect due to radioactivity. The decay of the nuclei emits heat. This deviates a little from standard thermodynamics, but it can be integrated into the models.
What poses more problems for us are questions of scale. In corium there is a separation between a solid metallic phase and a liquid metallic phase. Droplets and grains are of the order of a centimeter. But the interface between the two phases is of the order of a nanometer.
To simulate, we take thicker interfaces than in reality, making sure that the physical properties of interest, such as surface tension or interface kinetics, will be preserved. These properties shape the microstructures. If you don't capture them you can't properly represent what is happening.
What interests the CEA is to understand how, once the corium is formed, the heat diffuses. The mixture is not homogeneous. And there are heat transfers in the superstructure that is supposed to retain the corium. However, surface tension intervenes directly in the physics that allows us to predict these hot spots.
Our models are not the only ones at stake. Other specialties are mobilized. But you understand how these potentially very serious phenomena are also exciting problems for physicists.
* PMC: a joint research unit of CNRS, École Polytechnique - Institut Polytechnique de Paris